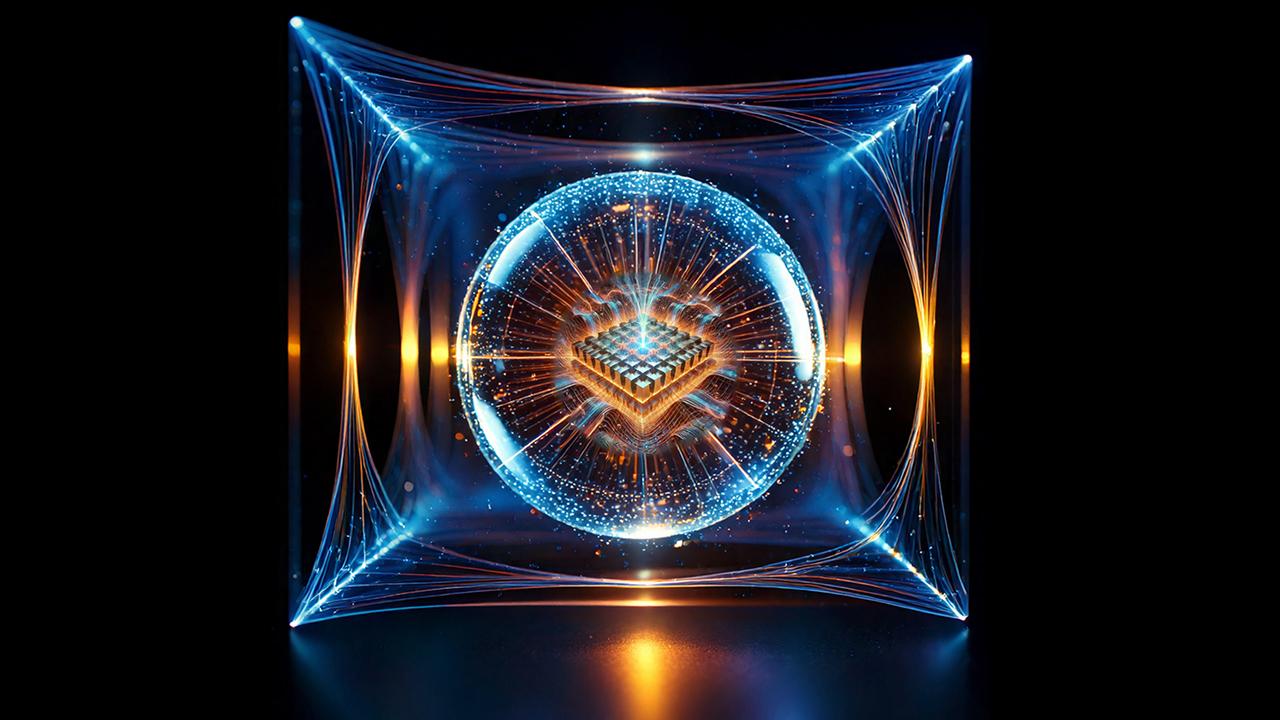
A collaboration led by ICTP has developed the first theoretical model of the fractional quantum Hall effect in a cavity. Published in Physical Review X, the study opens new avenues for understanding quantum matter by predicting new light-matter correlated states and lays a solid foundation for future theoretical and experimental investigations.
A striking example of quantum matter, the fractional quantum Hall effect occurs when a thin layer of strongly interacting electrons is cooled to just a fraction above absolute zero (-273 C), in the presence of a strong perpendicular magnetic field. In these materials the key feature of quantum mechanics—quantization—becomes observable at the macroscopic scale. In particular, the conductivity—a property describing how easily electrons move through the material—can only take up certain quantized values under these conditions, making fractional quantum Hall fluids very remarkable materials.
The fractional quantum Hall effect has been thoroughly studied over the years, and physicists have recently started confining these systems into cavities—tiny, controlled environments where light interacts strongly with matter—in search of new ways to better control these strange states of matter. A group of theoreticians, including researchers from ICTP, the International School of Advanced Studies (SISSA), the Italian National Research Council and Trento University, as well as the Indian Institute of Technology in Chennai, has developed a theoretical model that examines the behaviour of the fractional quantum Hall effect in such a cavity.
“Our model shows that the photons in the cavity interact in particular with an elusive type of quasiparticle—an emergent particle that captures the collective behaviour of strongly interacting electrons—which, in analogy with gravitational waves, has been called graviton. This interaction gives rise to hybrid light-matter correlated states, the graviton-polaritons,” says Zeno Bacciconi, the study’s first author and a PhD student at SISSA under the supervision of ICTP’s Marcello Dalmonte, a co-author of the paper.
“This new framework gives us a clearer understanding of how quantum Hall systems behave in the presence of a cavity. By predicting precise signatures of graviton-polaritons that should emerge from spectroscopy measurements, it also offers a way to search for these hybrid states in future experiments,” Dalmonte says.
The study also clarifies previous contrasting experimental findings, which in some cases suggested a breakdown of conductivity quantization when quantum Hall materials were confined in cavities. “Our model clearly shows that the cavity does not disrupt the fundamental character of the system,” says Bacciconi. “Further studies are needed to bridge the remaining gap between our theoretical predictions and experimental observations.”
The results provide important theoretical foundations for the study of fractional quantum Hall effects in cavities, a rapidly developing area of research. As physicists continue to explore ways to enhance and control quantum materials, this work will serve as a reference for further experiments and theoretical studies.
Link to the full article: https://link.aps.org/doi/10.1103/PhysRevX.15.021027