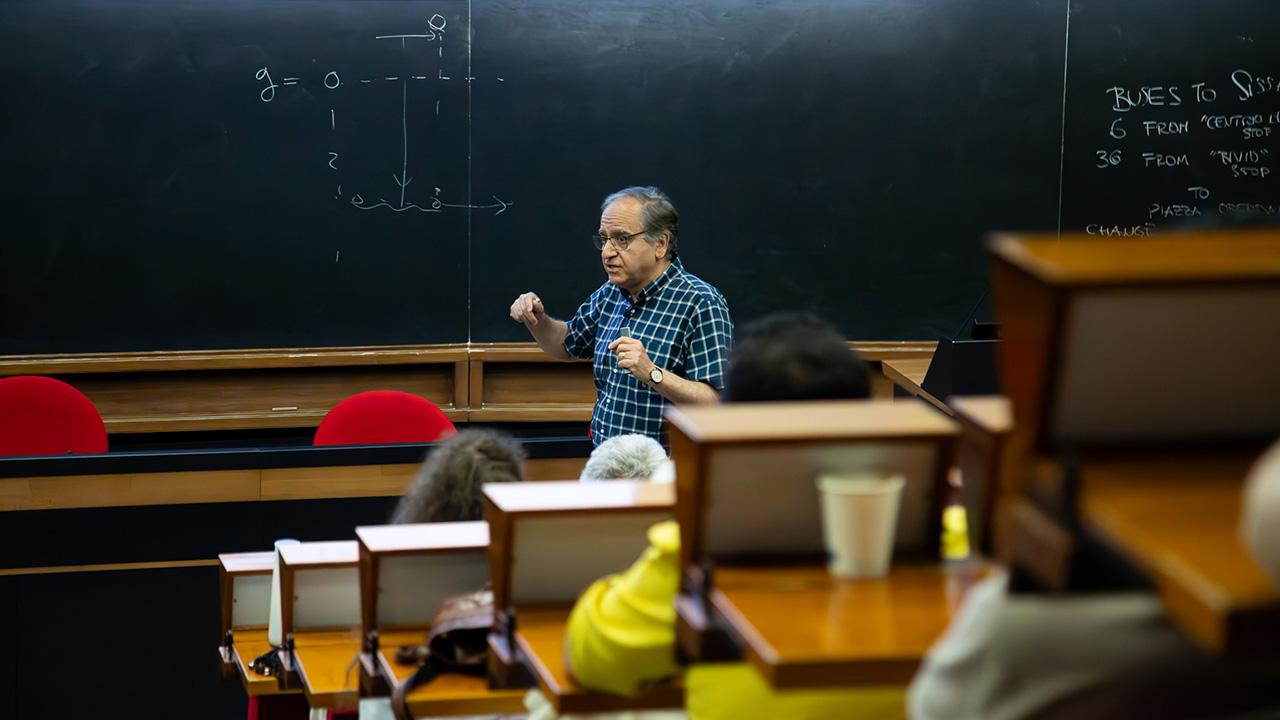
Cumrun Vafa is one of the most prominent world experts in string theory. The Hollis Professor of Mathematicks and Natural Philosophy in the Physics Department at Harvard University, and an ICTP Distinguished Staff Associate, he was among the speakers at the prestigious annual conference String Math 2024. The conference brings together mathematicians and physicists alike to discuss open problems in string theory, with an eye on the aspects of greatest interest to the mathematical community. ICTP had the honour to host this year’s edition, which took place from 10 to 14 June. It was attended by more than 200 participants from across the world and it featured some of the leading experts in the field.
Vafa kindly agreed to answer a few questions about string theory, his latest efforts to formulate testable predictions of the theory, the 2024 String Math conference and his experience with ICTP.
String theory is an ambitious framework that attempts to provide a consistent description of all the fundamental constituents of our universe, unifying general relativity with quantum mechanics. In this framework, fundamental particles are thought of as vibrational modes of one-dimensional objects called strings, or of two-dimensional objects, called branes. This has many implications, including the need to introduce extra spatial dimensions.
What attracted you to string theory?
I was interested in trying to learn how the fundamentals of the universe work, so I began studying particle physics and gradually, when string theory became a candidate for the unification of quantum mechanics with gravity, it naturally attracted my interest. I've been working on it since 1984, basically since I was a graduate student.
A central concept in string theory, and one that you have importantly contributed to, is duality. What is it and why is it so important?
Duality tells us that a physical system can be described in more than one way and that these different descriptions are related to one another. In other words, one way to get a handle on string theory is to work in specific regimes, characterised by extreme values of the parameters. Each one of these extreme regimes gives you a totally different picture of the system, although the underlying theory is the same. We call these descriptions dual to one another. They describe the same object, but in different regimes of parameters.
The power of duality is that it gives you a multitude of viewpoints on the same problem and, depending on which physical question you're asking, one regime or the other can give you the answer more or less effectively. This also means that you can tackle deep questions from different perspectives, each of which will tell you something that the others do not.
Your talk at the String Math 2024 conference was entitled "Cardy Formula and the Black Hole Entropy". Why are black holes, and particularly their entropy, so interesting to string theorists?
Black holes are perhaps the most enigmatic and interesting objects in quantum gravity. They are still mysterious, despite the fact that we have studied them for decades now. We know a lot of what happens outside black holes – we have even observed and photographed them – but we don't have a deep understanding of what goes on inside a black hole yet.
In the 1970s, Bekenstein and Hawking predicted some thermodynamic properties of black holes, including their entropy, implying that black holes do have an internal, microscopic structure. The fact that by using ideas from string theory we have been able to recover their results and provide a more detailed description of black holes [compared to the one provided by general relativity] was one of the first evidences that string theory is a complete theory of quantum gravity. This is why black holes became a central object in string theory and a litmus test of our understanding of quantum gravity.
What are you currently working on?
Lately, with my collaborators, I've been focusing on trying to summarize what the observable characteristics of a universe consistent with string theory would be.
Strikingly, we have been able to find some general principles of what it is that constitutes a consistent quantum theory of gravity, independently of all the possible choices that one can make. We have found that these general principles can be combined with observations that we already have – such as the value of the cosmological constant or of dark energy – to make new predictions that can actually be tested.
What are some examples of predictions from string theory that can be compared with observations?
An example of a simple qualitative prediction that one can make and that has actually already been confirmed is that of the weakness of gravity. We found that in any universe compatible with string theory, gravity can never be anything but the weakest force. And this of course agrees with observations because we know very well that in our universe gravity is the weakest force.
Using similar ideas, we can go much further. By applying these same ideas to dark energy, what we have learnt is exactly the connection between duality and extreme parameters that I was talking about earlier. As I explained, when you take the parameters of your theory to their extreme values, you get one perspective of that theory. Such perspective, however, comes with some extra degrees of freedom, in the form of light particles.
Dark energy, which in fundamental units of physics is as small as 10-122, is a very good example of an extreme quantity. One might then wonder why we have not seen its associated light particles. Together with my collaborators, we claim that in the case of dark energy light particles are found in dark matter. In other words, dark energy being extremely small leads to the existence of dark matter. In this way string theory provides a unified picture of dark matter and dark energy.
There is more. The observed value of dark energy leads to the prediction that at least one of the extra dimensions should be as big as a micron. This is a tiny scale, but not so tiny after all - it’s bigger than an atom.
We know that in a three-dimensional space, the gravitational force between two masses is inversely proportional to the square of the distance. One can show that if you add one extra dimension, the gravitational force is inversely proportional to the cube of the distance. These effects are not visible if one looks at distances larger than the extra dimension, but we expect them to become visible at shorter distances. In particular, when measuring the gravitational force as a function of decreasing distances, we expect to see a switch in the power law at distances of the order of one micron, with the exponent slowly going from 2 to 3. We therefore expect the gravitational attraction to increase faster at distance smaller than a micron.
So far it has been possible to measure that the inverse square law holds at distances up to about 30 microns. A new round of experiments performed in Vienna is testing what happens at distances of about ten microns. As we become able to test smaller and smaller scales, we could potentially see something very interesting happening at the scale of one micron and that’s very exciting.
Thirteen years have passed since the first String Math conference was organised in 2011, and you have attended many editions. What would you say this closer collaboration between physicists and mathematicians has brought to the field?
This has been a very fruitful interaction, for both maths and physics, and I think that great progress has been made in multiple directions. The ones of mathematicians and of physicists are two clearly distinct communities. Some of the topics that we discuss are very mathematical, some of them are very physical and one can clearly see how each community strives to understand the other’s perspective, in a way that helps both fields get a deeper understanding of the problems at hand.
What main message or question are you taking home from this conference?
I'm going home reassured to see that there is a great community of young researchers that are going to keep this field alive for many, many decades to come. In this particular occasion I have been pleasantly surprised by the high quality of the research being done right now. Even more so, I have been especially pleased to see that there are so many young people contributing to the development of these ideas. It makes me very happy that this field is progressing rapidly thanks to the contributions of all these talented people. That’s extremely exciting and I'm very optimistic for the future of our field, given what I saw both in terms of the quality of the talks and of the sheer number of people interested in this topic. This has probably been one of the biggest String Math conferences that I have been to and I think this is very promising.
As you know, ICTP is celebrating its 60th anniversary this year and you have known the Centre for a very long time. What would you say ICTP has done for the global scientific community?
I came to ICTP for the first time in 1984, when I was a graduate student. Already back then, I thought that ICTP was a magical place, and I found it pleasantly shocking that such a research centre, where top notch science was being developed and made available to all, especially to those who would have not have had access to it in their home countries, existed.
Four decades since I first came to ICTP, I experience the same pleasant astonishment now, when I look at how this place has been stronger than ever. ICTP is a beacon of hope for the development of science and a meeting place for international researchers. The String Math conference that just took place is just an example of how, in addition to the work that ICTP does in collaboration with scientists from developing countries, it also is a great place for high-level science. I hope it receives all the support that it needs to continue attracting great researchers from across the world.
Cumrun Vafa received his B.S. in math and physics from MIT and a Ph.D. in theoretical physics from Princeton University. He has received numerous prizes and recognition for his work on theoretical physics, including the 2017 Breakthrough Prize in Fundamental Physics, the 2008 Dirac Medal of ICTP and prizes for his work on mathematical physics from the American Mathematical Society, as well as the American Physical Society. He is a member of the National Academy of Sciences as well as the American Academy of Arts and Sciences.